Exo Gas Composition Changes, Part 1: Production
Exothermic gas undergoes a few metamorphoses from the time it is produced to the time it is cooled down after use. Explore the transformations that occur within the combustion chamber to discover the impact these phases can have on the heat treatment atmosphere of your workpieces.
This Technical Tuesday article was composed by Harb Nayar, president and founder, TAT Technologies LLC. It appears in Heat Treat Today's August 2023 Automotive Heat Treating print edition.
Background
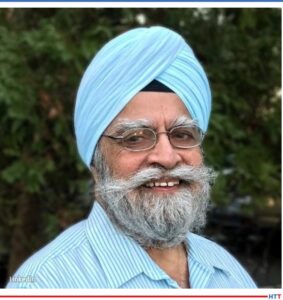
President and Founder
TAT Technologies LLC
Source: LinkedIn
Exothermic gas, more commonly referred to as Exo gas, is produced by partial combustion of hydrocarbon fuels with air in a well-insulated reaction or combustion chamber at temperatures well above 2000°F. Immediately after they exit the combustion chamber, the reaction products are cooled down using water to a temperature below ambient temperature to avoid condensation. The typical dew point of the cooled down Exo gas is about 10°F above the temperature of the water used to cool down. The cooled down Exo is then delivered to the heat treat furnaces where it gets reheated to the operating temperatures between 300°F and 2100°F.
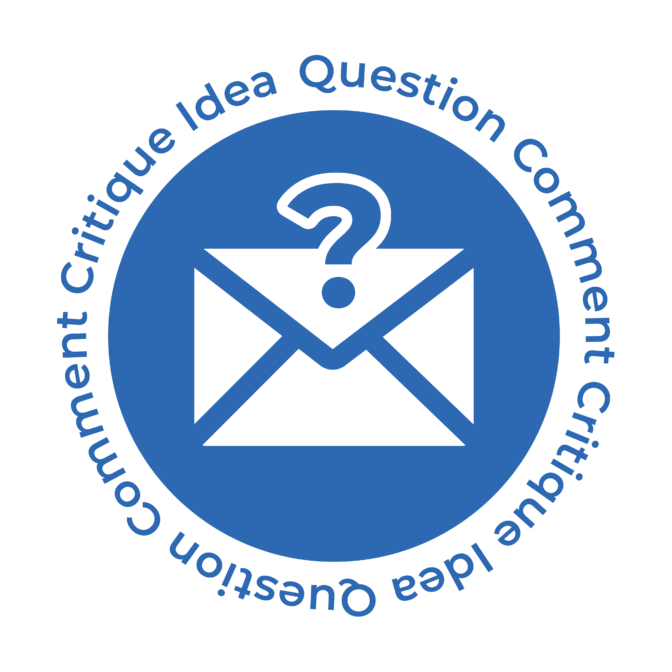
A simplified schematic flow diagram of Exo gas production followed by its cool down below ambient temperature and its final use in heat treat furnaces is shown in Figure 1.
The following aspects of the Exo gas production are clear from Figure 1:
- There is lot of energy lost out of the reaction chamber.
- There is additional heat lost during cooling using water.
- A good deal of water is used for cooling.
- The cooled down Exo gas is re-heated to the process temperature in heat treat furnaces.
Exo gas has been predominantly used and is still being used as a source of nitrogen rich atmosphere for purging, blanketing, and mildly oxide reducing applications in the heat treat and metal working industries.
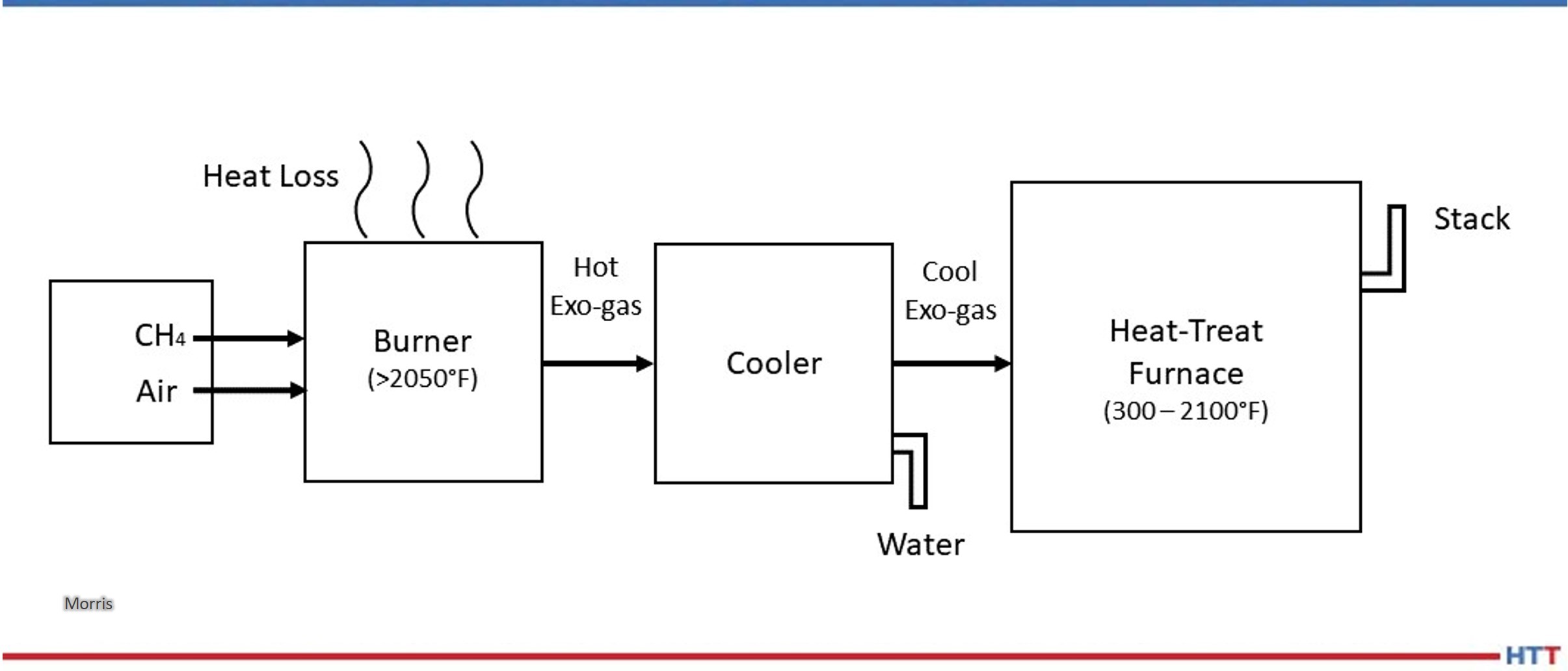
Source: Morris, “Exothermic Reactions,” 2023
Examples of applications:
- Brazing
- Annealing
- Hardening
- Normalizing
- Sintering
- Tempering, etc.
Examples of materials:
- Irons
- Steels
- Electrical steels
- Copper
- Copper-base alloys
- Aluminum
- Jewelry alloys
Examples of product sizes and shapes:
- Tubes
- Rods
- Coils
- Sheets
- Plates
- Components
- Small parts, etc.
Exo is the lowest cost gas used in furnaces operating at temperatures above about 700°F to keep air out and provide a protective atmosphere with some oxide reducing potential to the materials being thermally processed.
There are two types of Exo gases: lean Exo gas, with mostly nitrogen and carbon dioxide and very little hydrogen, and rich Exo gas, with a little less nitrogen and carbon dioxide and substantially more hydrogen and some carbon monoxide. Typical compositions are given below:
- Lean Exo: 80–87% Nitrogen; 1–2% Hydrogen; 2–4% H20; 1–2% CO; 10–11% CO2
- Rich Exo: 70–75% Nitrogen; 9–12% Hydrogen; 2–4% H20; 7–9% CO; 6–7% CO2
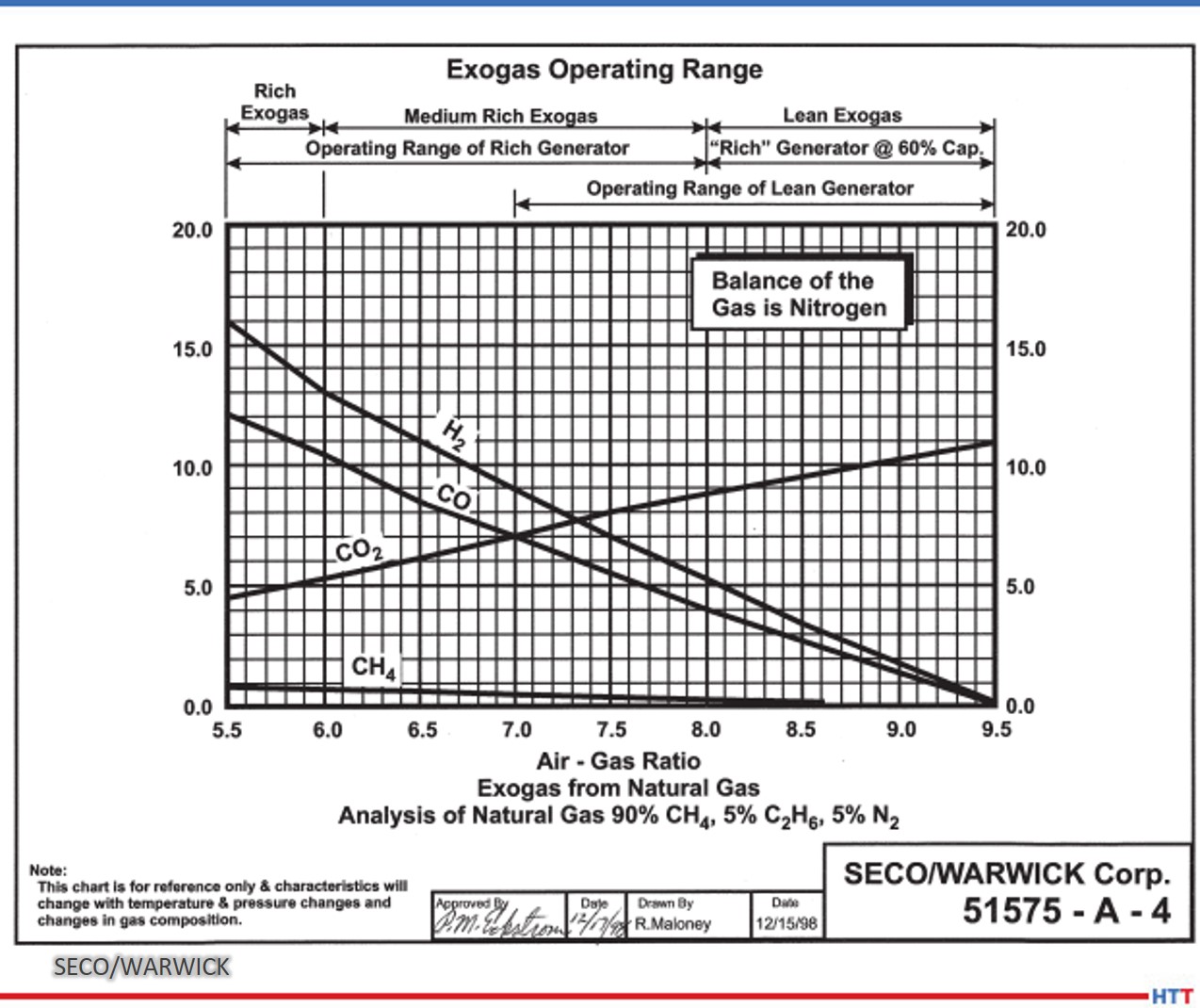
Source: SECO/WARWICK
Figure 2 shows graphs of Exo gas composition at various air to natural gas ratios. H2, CO, and residual CH4 decreases with increasing air to natural gas ratio whereas CO2 goes in the opposite direction. H20 content not shown in the graphs is typically in the 2–4% range depending upon the temperature and cooling efficiency of the cooling system. N2 is the balance which increases with increasing air to natural gas ratio.
The generator designs to produce lean and rich Exo gases are slightly different as shown in the schematic flow diagrams below in Figures 3 and 4.
Objective
This paper will demonstrate a simplified software program (harb-9US) developed recently by TAT Technologies LLC that can easily calculate the reaction products composition, temperature, exothermic energy released, various ratios, and final dew point for various combinations of air and fuel flows entering the reaction chamber at a predetermined temperature and pressure.
The data presented in this paper is under thermodynamically equilibrium conditions only, captured when the reaction is fully completed. It does not tell how long it will take for the reaction to reach completion. However, it can be safely said that reactions are completed relatively fast at temperatures above about 1500°F and very slow at temperatures below about 1000°F. The current software program uses U.S. units: flow in SCFH, pressure in PSIG, temperature in degrees Fahrenheit, and heat as enthalpy in BTU.
The composition of the Exo gas for a fixed incoming air to hydrocarbon fuel ratio changes from production in the combustion chamber to the cool down equipment to bring the Exo gas to below the ambient temperature and finally into the furnace where the material is being heat treated.
Understanding the changes in gas composition from Step 1 (Production in the Combustion Chamber) to Step 2 (Cool Down to Ambient Temperature) to Step 3 (At Temperature of Heat Treated Part) can help to improve the composition, quality, and control of Exo gas that will surround the metallic products being heat treated in the furnace.
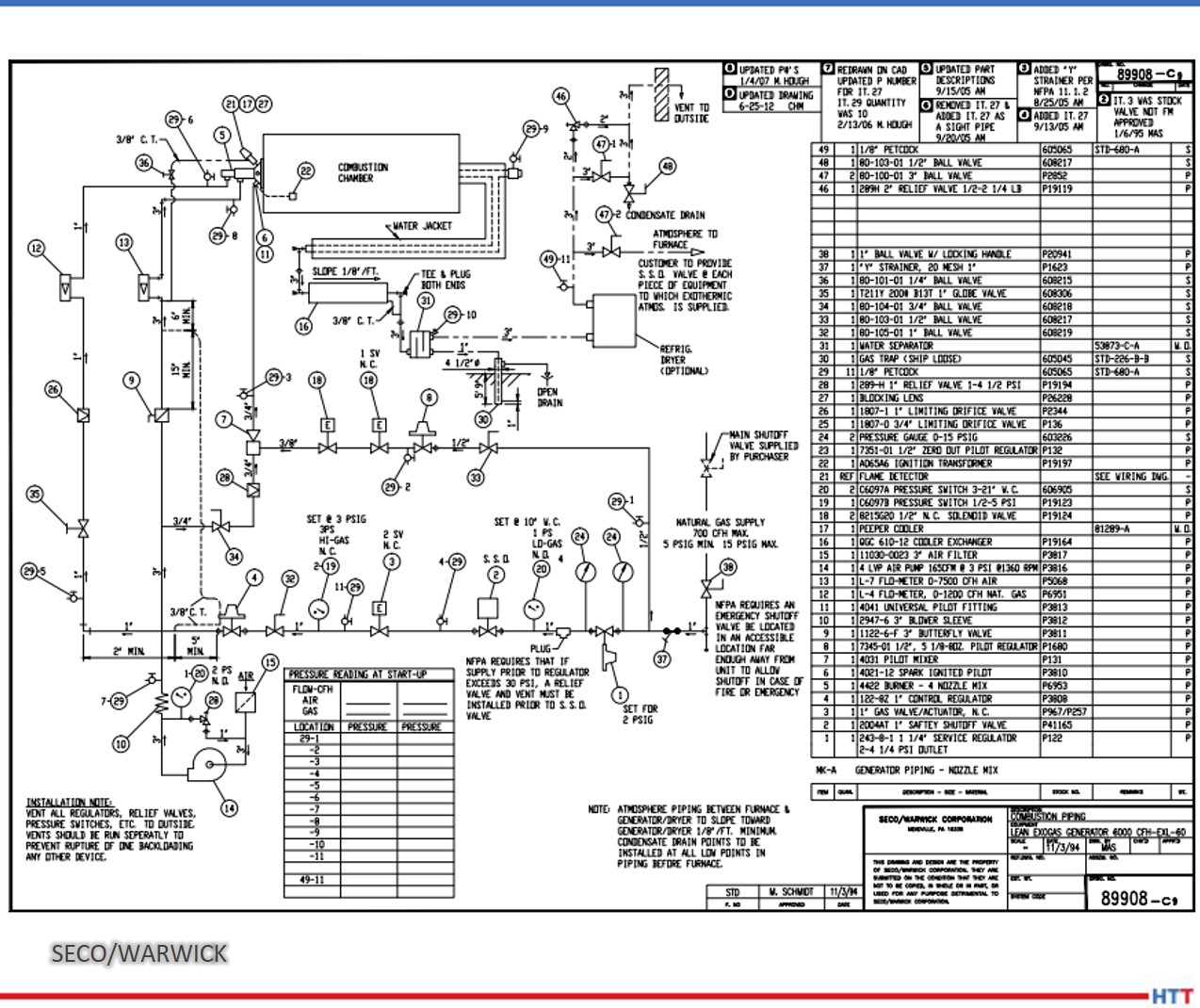
Source: SECO/WARWICK
Step 1: Composition of Exo Gas as Produced in the Combustion Chamber
Table A shows the Exo gas compositions as generated within the combustion chamber at various air to natural gas ratios supplied at 100°F and 0.1 PSIG. In these calculations natural gas composition is assumed as 100% CH4 and air is assumed as 20.95% oxygen and balance nitrogen. CH4 is fixed at 100 SCFH and air flow is varied to give air to natural gas ratios between 9 and 6. Typically a ratio of 9 is used for lean Exo and 7 is used for rich Exo applications. Other ratios are used in some special applications.
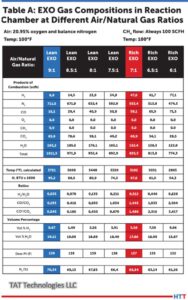
Source: TAT Technologies LLC
The following key conclusions can be made from Table A as one moves from air to natural gas (CH4) ratio of 9 down to 6:
- The peak temperature in the reaction chambers goes from a high of 3721°F down to low of 2865°F. Because of high temperatures, good insulation around the combustion chamber is a must. A significant portion of the exothermally generated energy within the reaction chamber is lost to the surroundings.
- There is no residual CH4 in the Exo gas composition at these high temperatures. There is no soot (carbon residue) under equilibrium conditions.
- H20 content in the natural gas (CH4) gas in the reaction chamber is very high — from high of 19.11% to low of 15.87%. These correspond to dew point 139°F to 132°F — well above the ambient temperature. Because of the very high dew point, the Exo gas coming out of the reaction chamber must be cooled down below the ambient temperature to remove most of the H20 in the Exo gas to avoid any condensation in the pipes carrying the Exo gas toward the furnace and into the
furnace. - H2% changes significantly from 0.67% to 9.96%.
- The oxide reducing potential (ORP) as measured by H2/H20 ratio changes from a very low of 0.035 to 0.628. ORP in the reaction chamber is overall quite low because of high percentage of H20.
- Nitrogen content varies from 70.34% to 61.26% of the total Exo gas in the reaction chamber.
- Exothermic heat generated varies from 95.3 MBTU to 54.34 MBTU — it gradually becomes a less exothermic reaction. Gross heating value of CH4 (at full combustion) is 101.1 MBTU/100 cubic foot of CH4.
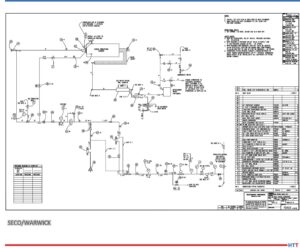
Source: SECO/WARWICK
Question: What happens to the composition of Exo gas as it cools from peak temperature in the combustion chamber to different lower temperatures after it exits from the combustion chamber?
Answer: It changes a LOT, assuming enough time is provided to reach its equilibrium values during cooling down to any specific temperature. Whenever there is a mixture of gases, such as CH4, H2, H20, CO, CO2,O2, N2, there are a variety of reactions going on between the constituents in the reactant gases to produce different combinations of gas products and heats (absorbed or liberated) at different temperatures. The most popular and well-known reactions are:
- Partial Oxidation Reaction: CH4+ 1/2O2 → CO + 2H2 — exothermic. The reaction becomes more exothermic as O2 increases from 0.5 to 2.
- Water Gas Shift Reaction: CO + H20 → CO2 + H2 — slightly exothermic. It usually takes place at higher temperatures faster. A catalyst in the reaction chamber can help to lower the high temperature requirement. There are many catalysts. Commonly used are either Ni or precious metals.
- Steam Reforming Reaction: CH4 + H20 → CO + 3H2 — highly endothermic.
- CO2 Reforming Reaction: CH4 + CO2 → 2CO + 2H2 — endothermic.
All of these reactions have different degrees of influences from changes in temperature. One could say that the final equilibrium composition of the Exo gas is a continuously moving target as temperature changes. Only the N2 portion stays constant. One can make the following generalized statements covering a broad range of Exo gases (lean and rich) in the reaction chamber:
a) N2 content does not change. It remains neutral at all temperatures.
b) H2 content decreases with increasing temperature.
c) H20 (vapor) content increases with increasing temperature.
d) CO content increases with increasing temperature.
e) CO2 content decreases with increasing temperature.
f) Residual CH4 decreases with increasing temperature.
g) Soot decreases with increasing temperature.
h) Catalysts facilitate the speed of reactions at any temperature.
Conclusion
Exo gas composition changes during its time in the combustion chamber. Reaction products composition, temperature, exothermic energy released, various ratios, and final dew point are all items that need to be taken into consideration to protect the metallic pieces that will be heat treated in the resulting atmosphere. Part 2 will demonstrate this principle and discuss Step 2 (Cool Down to Ambient Temperature) and Step 3 (At Temperature of Heat Treated Part).
About the author:
Harb Nayar is the founder and president of TAT Technologies LLC. Harb is both an inquisitive learner and dynamic entrepreneur who will share his current interests in the powder metal industry, and what he anticipates for the future of the industry, especially where it bisects with heat treating
For more information:
Contact Harb at harb.nayar@tat-tech.com or visit www.tat-tech.com.
References:
Herring, Dan. “Exothermic Gas Generators: Forgotten Technology?” Industrial Heating, 2018. https://digital.bnpmedia.com/publication/m=11623&i=534828&p=121&ver=html5.
Morris, Art. “Exothermic Reactions.” Industrial Heating (June 10, 2023), https://www.industrialheating.com/articles/91142-exothermic-atmospheres.
Find heat treating products and services when you search on Heat Treat Buyers Guide.com
Exo Gas Composition Changes, Part 1: Production Read More »