Dive into the role and benefits of HIP and HPHT™ in the space industry, highlighting how these key processes are shaping the future of space applications.
This Technical Tuesday article by Andrew Cassese, applications engineer, Quintus Technologies was originally published in Heat Treat Today’s March/April 2024 Aerospace print edition.
The realm of space exploration and technology is rapidly evolving, pushing the boundaries of what’s possible in engineering and material science. Among the key players in this revolutionary change are hot isostatic pressing (HIP) and High Pressure Heat Treatment™ (HPHT™). These processes have become indispensable in manufacturing components that can withstand the harsh conditions of space. In this demanding environment, the longevity and reliability of components are paramount.
Reducing Risk
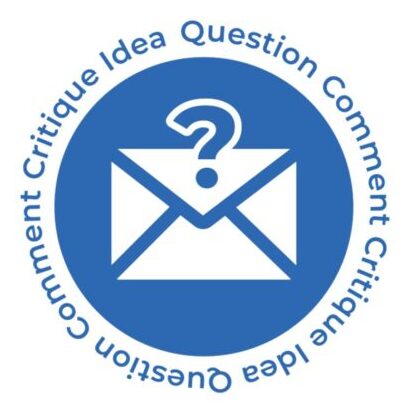
Space missions have put increasing focus on the need to minimize risk and improve mission safety. Some well-documented, safety-related events include:
- Outer space
- Soyuz 11 decompression in 1971
- Earth’s atmosphere
- Soyuz 1 parachute failure in 1967
- X-15 controls failure in 1967
- Space Shuttle Challenger launch
booster failure in 1986 - Space Shuttle Columbia re-entry
disaster in 2003
Structural integrity is therefore in focus for every single component involved in space missions, with exacting demands on quality and function. Material failure is not an option, and therefore component qualification is one of the main areas of focus. Predictable properties that are reliable and with minimal variation are critical for mission safety. Hot isostatic pressing helps to guarantee this by reducing the spread and variation in mechanical properties.1 It works to do this by using high temperatures and pressures to close internal defects in mission critical parts after casting or additive manufacturing. This increases the density of components and gives them a more anisotropic microstructure which in turn results in more consistent mechanical properties.2
What Properties Are Most Important
The harsh environment of space demands components with exceptional properties. They must withstand extreme temperatures, resist radiation, endure vacuum pressures, and cope with mechanical stress from vibrations and accelerations. HIP processing plays a pivotal role in this, enhancing material properties to meet these challenges. Space manufacturers also must think about thermal expansion/contraction due to temperature variations, compressive stresses, irradiation, and space debris. All of these can affect mission success and can ultimately prevent loss of life, see Figure 1.

Through HIP, components gain increased fatigue life, improved ductility, and enhanced fracture toughness, which are crucial for surviving in space.
Common Materials and HIP Processing Requirements
Materials commonly processed by HIP for space applications include titanium, aluminum alloys, nickel-based superalloys, refractory alloys, shape memory alloys, and ceramics. High-strength aluminum and titanium alloys are used due to their high strength to weight ratio which is key for space missions to conserve fuel efficiency, increase payload capacity, and improve maneuverability.3 Nickel-based superalloys are used in exhaust valves and turbine rotors due to their exceptional creep resistance properties at high temperatures. Refractory alloys like Nb-C103 and TZM are used in high-performance rocket nozzles because of their high melting point and excellent strength at high temperatures. Newer shape memory alloys developed by NASA can recuperate their original shape when heating above specific critical temperatures, and their applications are expanding beyond just actuators.4
As new alloys and materials are developed in the space industry, certifications and standards are necessary for their adoption. HIP effectively eliminates porosity in these materials, ensuring structural integrity and performance under the extreme conditions of space. This means HIP recipes need to be developed and optimized for materials to be tested with their greatest potential in mind.
Challenges in Processing Space Components
Processing components for space via HIP is not without its challenges. Th e extreme conditions required for HIP, including high temperatures and pressures, demand robust and sophisticated equipment. Quintus Technologies applications centers utilize a graphite furnace capable of heating to 3632°F (2000°C), while maintaining pressures of 30,000 psi (200 MPa). The process requires precise control to ensure uniformity of properties across the component. Specifically, the graphite uniform rapid cooling© (URC©) furnace can maintain temperature uniformity while controlling to a specified cooling rate.
Another challenge with processing space components in HIP can be oxidation of parts in the HIP furnace atmosphere. Niobium, for example, can suffer from substantial oxidation at elevated temperatures. In practice, tantalum foil is typically used to wrap the niobium components during HIP and to prevent oxidation from any residual moisture in the argon atmosphere. New products, like the Quintus Purus©, can reduce the amount of oxidation seen on parts aft er HIP while saving valuable time and resources by not having to wrap parts with getter materials like stainless steel, titanium, or tantalum.
Ongoing Research and Unknowns
Collaborations with universities and national labs on projects at low TRLs will help set the foundation for HIP in the space industry. Quintus Technologies, through its application centers, is actively engaged in research to further enhance the capabilities of HIP for space applications. Optimizing the HIP process to reduce costs and improve efficiency through HPHT is one area where the company has already found success, see Figures 2 and 3.


The HPHT process can combine stress relief, solution annealing, HIP, and aging into one cycle. Aft er a ramp up in pressure and temperature, the part is held for a specified amount of time before being rapidly cooled in the URC furnace. Aft er this, the temperature of the machine can be brought up to the aging temperature of the material for the completion of an in situ heat treatment.
A Space Case – Launcher Engine-2 Rocket Engine

One application of this is on the Launcher Engine-2 (E-2) rocket engine.
Quintus Technologies, EOS Group, and Launcher worked together to develop a tailored HPHT cycle for Launcher’s 3D printed E-2, first vetted out in an applications center at small scale. The powder alloy in question, CuCrZr, was developed by EOS and printed on an AMCM M4K machine. EOS compared CuCrZr to the NASA alloy of GRCop-42 and found that the CuCrZr alloy was a more economically viable solution for thermal applications with lower strength requirements, see Table 1. The rapid cooling at 200°C/min in the QIH 122 URC furnace at Aalberts surface technologies allowed the team to HIP and solution heat treat the CuCrZr combustion chamber in a single step. The aging treatment was also performed in the QIH 122 directly aft er the solution.5
In October 2020, a full-scale test firing of the E-2 injector and combustion chamber was conducted at the Launcher NASA Stennis Space Center test stand. On April 21, 2022, Launcher’s E-2 liquid rocket engine was able to demonstrate full thrust. Continued tests from Launcher have been successful with performance boost testing
and the first fully integrated engine was ready for shipping on October 12, 2023.6

Conclusion
As humanity reaches further into the cosmos, the role of HIP and HPHT in manufacturing space-bound components becomes increasingly significant. These processes not only enhance the essential properties of materials for space applications but also address the unique challenges of manufacturing for an environment as hostile as space. With ongoing research and development, HIP and HPHT continue to evolve, promising to unlock new possibilities in space exploration and technology, and their contribution will ensure the success of space missions, safeguarding the lives of those who venture into the final frontier.

References
[1] Dominik Ahlers and Thomas Tröster, “Performance Parameters and HIP Routes for Additively Manufactured Titanium Alloy Ti6Al4V. EuroPM,” 2019. https://www.semanticscholar.org/paper/Performance-Parameters-and-HIP-Routes-fortitanium-
Ahlers-Tr%C3%B6ster/faeb46e6eb8ef3e30bc00b91cd1bd8a7c0619200.
[2] Jake T. Benzing et al., “Enhanced strength of additively manufactured Inconel 718 by means of a simplified heat treatment strategy,” Journal of Materials Processing Technology 322, (December 2023). https://www.sciencedirect.com/science/article/abs/pii/S0924013623003424?via%3Dihub.
[3] “Engineering Materials for Space Building Stronger Lighter Structures,” Utilities One, last modified November 2023. https://utilitiesone.com/engineering-materials-for-space-building-stronger-lighter-structures.
[4] Girolamo Costanza and Maria Elisa Tata, “Shape Memory Alloys for Aerospace, Recent Developments, and New Applications: A Short Review,” Materials (Basel) 13, no. 8 (April 2020): 1856. https://www.ncbi.nlm.nih.gov/pmc/articles/PMC7216214/.
[5] Mahemaa Rajasekar, “Processing Copper Alloys with Powder Bed Fusion,” LinkedIn, last modified November 2022. https://www.linkedin.com/pulse/processing-copper-alloys-dmls-technology-mahemaarajasekaran/.
[6] LAUNCHER (@launcher), “The first fully integrated E-2 engine is ready for shipping to @NASAStennis for our upcoming full engine test campaign later this year. E-2 is a 22,000 lb. (10 ft) thrust LOX/Kerosene,” X post, October 12, 2023. https://twitter.com/launcher/status/1712636548997607752.
About the Author

Technologies
Andrew Cassese is an applications engineer at Quintus Technologies. He has a bachelor’s degree in welding engineering from The Ohio State University.
For more information: Read J Shipley, “Hot Isostatic Pressing in Space – Essential Technology to Ensure Mission Safety,” 2020. Contact Andrew at andrew.cassese@quintusteam.com.